Leggett at KTH: Revising How We Understand the Arrow of Time
NOBEL PHYSICIST
At the AlbaNova Colloquium, Sir Anthony Leggett, the 2003 Nobel Physics Prize winner, talks about the science of quantum mechanics, looking at how research into the very strange behaviour of atomic particles is beginning to deliver new technologies — even before the fundamental principles are fully understood.
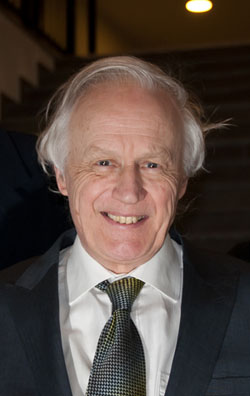
There’s a certain aura about Nobel Prize winners. Having earned the ultimate peer recognition for scientific achievement, they tend to be self-confident, humble and personable, but it often appears that social chitchat is more an obligation to endure than a welcome opportunity accompanying membership in one of the world’s most elite clubs. Professor Anthony James Leggett, the 73-year-old British Nobel Physics Prize winner who visited KTH February 16, fits neatly into this admittedly unscientific stereotype.
Of the 830 individual recipients of Nobel prizes since 1901, only about 250 are alive today. And having had the opportunity to meet only a handful of those busy few who still work, travel and lecture, my sample size is far too small to justify the sweeping generalisation above. Let’s just say that, like others in this small circle, Professor Leggett seems more at home with his arcane equations than he is with inquisitive reporters.
Introducing myself when he arrives outside the lecture hall a few minutes before his address to the AlbaNova Colloquium, I tell him that one of his hosts, KTH Professor David Haviland, had committed a physicist’s Freudian slip in an email the evening before. “David wrote that he told you I'd be here to ask a few questions and take some photons. That’s actually a technically accurate description of what a camera does, even if most people don’t usually describe it so literally.”
Professor Leggett — or Sir Anthony as he’s also known since being knighted by Queen Elizabeth in 2004 — laughs heartily at the attempted ice-breaker, but I get the feeling that he’s not entirely comfortable being the centre of attention as well-wishers approach to shake his hand and exchange a few words of greeting. Inside the lecture hall, however, armed with a microphone and a projector before an expectant audience comprised mostly of physics researchers, it becomes clear that this is his natural element. It’s a dignified, courtly gentleman who clears his throat to begin, but an intellectually dominating researcher who takes command of the room for the next hour.
Professor Leggett shared the 2003 Nobel Prize for his work in low temperature physics — in particular for his research on superfluid helium — but his AlbaNova lecture delves into quantum mechanics and the mysterious phenomenon known as “entanglement,” one of the many other areas of physics to which he has made groundbreaking contributions.
To envision this bizarre concept, imagine two atoms, prepared by an experimenter to spin in opposite directions like toy tops turning either clockwise or counterclockwise. But the experimenter doesn’t know which atom spins in which direction — in fact, the spin of each atom is actually indeterminate until it is observed. This means that, for each atom, there is a 50 per cent probability that it is spinning in either direction. By the weird but experimentally proven rules of quantum mechanics, when the spin of one atom is measured, the other atom instantaneously assumes the opposite spin even if the atoms are light-years apart. The act of observing one atom changes the state of an entangled atom regardless of distance and without any passage of time. Science has shown the phenomenon to be true, but no one can say why or how.
“My gut instinct is that, if the solution ever comes, it will require a drastic revision of how we understand the arrow of time,” Leggett says. “It’s something we really don’t understand. At least I don’t.”
But he goes on to describe how practical applications are on the horizon even before Nature reveals the secrets of quantum entanglement. Perhaps most promising is secure-in-principle cryptography. Leggett mentions commercial systems already on the market that use photons transmitted by optical fibre to deliver the coded keys to unlock an encrypted message by defining digital bits in terms of quantum spin states. An eavesdropper listening in on the transmission between sender and receiver (a common scenario known to cryptographers as a “man-in-the-middle attack”) would have to observe the spin states to read the keys, but that very act of observation changes the spin state at the intended receiver’s end, rendering the message unintelligible.
“If Alice sends a message to Bob and Eve listens in, she’ll spoil everything and Bob and Alice will know immediately that their communication is compromised,” Leggett says. “This method is almost certainly under development in ways that we don’t know anything about at various high-security laboratories around the world.”
Leggett went on to explain how entanglement also comes into play in the emerging field of quantum computing, where every part of the computation is correlated to every other part. Unlike the simple zero-one transistor technology of the computer on your desk, a quantum computer represents data as quantum bits, or qubits, each of which can represent a one, a zero, or any theoretically possible state simultaneously. When measured, the qubit gives a result corresponding to only one of its possible configurations. “In theory, this leads to the possibility of massively parallel computing,” Leggett says. “A calculation that would take a classical computer more time than the age of the universe to solve could be done in a few minutes on a quantum computer.”
For most of us, the age of the universe wouldn’t be enough time to grasp how measuring an atom’s spin on earth can magically define the state of an atom on the other side of the galaxy. We’ll just have to leave that to the rare minds of Nobel laureates.
By Kevin Billinghurst | kb2@kth.se