New theory explains how Earth’s inner core remains solid despite extreme heat
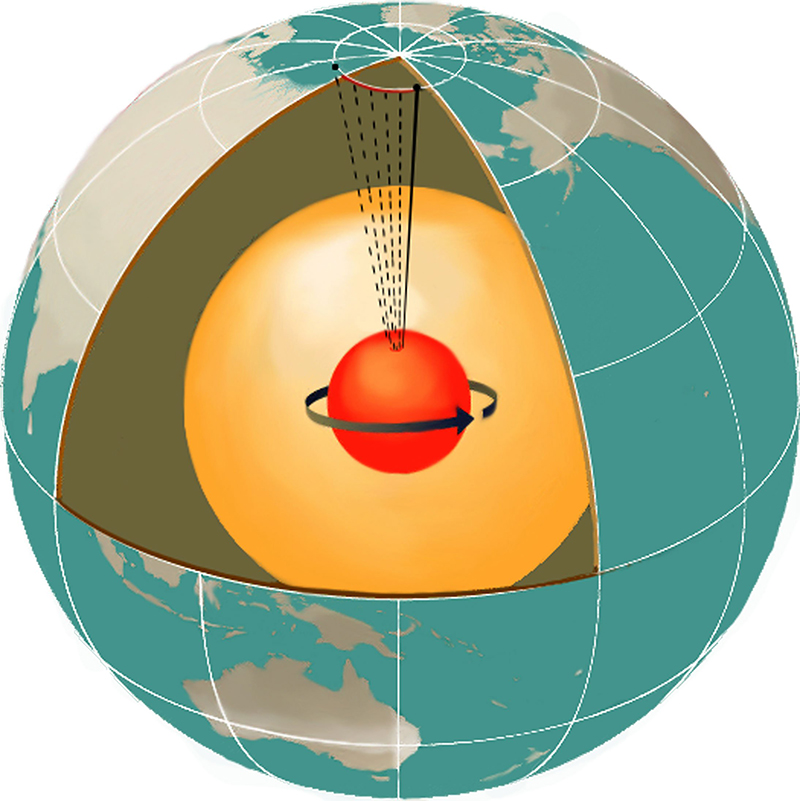
Even though it is hotter than the surface of the Sun, the crystallized iron core of the Earth remains solid. A new study from KTH Royal Institute of Technology may finally settle a longstanding debate over how that’s possible, as well as why seismic waves travel at higher speeds between the planet’s poles than through the equator.
Spinning within Earth’s molten core is a crystal ball – actually a mass formation of almost pure crystallized iron – nearly the size of the moon. Understanding this strange, unobservable feature of our planet depends on knowing the atomic structure of these crystals – something scientists have been trying to do for years.
As with all metals, the atomic-scale crystal structures of iron change depending on the temperature and pressure the metal is exposed to. Atoms are packed into variations of cubic, as well as hexagonal formations. At room temperatures and normal atmospheric pressure, iron is in what is known as a body-centered cubic (BCC) phase, which is a crystal architecture with eight corner points and a center point. But at extremely high pressure the crystalline structures transform into 12-point hexagonal forms, or a close packed (HCP) phase.
At Earth’s core, where pressure is 3.5 million times higher than surface pressure – and temperatures are some 6,000 degrees higher – scientists have proposed that the atomic architecture of iron must be hexagonal. Whether BCC iron exists in the center of the Earth has been debated for the last 30 years, and a recent 2014 study ruled it out, arguing that BCC would be unstable under such conditions.
However, in a recent study published in Nature Geosciences , researchers at KTH found that iron at Earth’s core is indeed in the BCC phase. Anatoly Belonoshko , a researcher in the , says that when the researchers looked into larger computational samples of iron than studied previously, characteristics of the BCC iron that were thought to render it unstable wound up doing just the opposite.
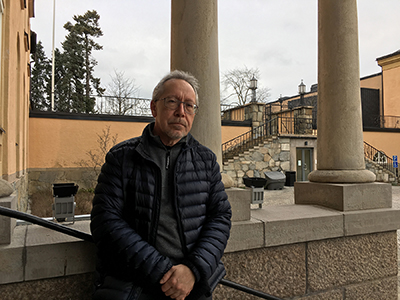
“Under conditions in Earth’s core, BCC iron exhibits a pattern of atomic diffusion never before observed,” Belonoshko says.
Belonoshko says the data also shows that pure iron likely accounts for 96 percent of the inner core’s composition, along with nickel and possibly light elements.
Their conclusions are drawn from laborious computer simulations performed using Triolith, one of the largest Swedish supercomputers. These simulations allowed them to reinterpret observations collected three years ago at Livermore Lawrence National Laboratory in California. “It appears that the experimental data confirming the stability of BCC iron in the Core were in front of us – we just did not know what that really meant,” he says.
At low temperature BCC is unstable and crystalline planes slide out of the ideal BCC structure. But at high temperatures, the stabilization of these structures begins much like a card game – with the shuffling of a “deck”. Belonoshko says that in the extreme heat of the core, atoms no longer belong to planes because of the high amplitude of atomic motion.
“The sliding of these planes is a bit like shuffling a deck of cards,” he explains. “Even though the cards are put in different positions, the deck is still a deck. Likewise, the BCC iron retains its cubic structure.”
Such a shuffling leads to an enormous increase in the distribution of molecules and energy – which leads to increasing entropy, or the distribution of energy states. That, in turn, makes the BCC stable.
Normally, diffusion destroys crystal structures turning them into liquid. In this case, diffusion allows iron to preserve the BCC structure. “The BCC phase goes by the motto: 'What does not kill me makes me stronger',” Belonoshko says. “The instability kills the BCC phase at low temperature, but makes the BCC phase stable at high temperature.”
He says that this diffusion also explains why the Earth’s core is anisotropic – that is, it has a texture that is directional – like the grain of wood. Anisotropy explains why seismic waves travel faster between the Earth’s poles, than through the equator.
"The unique features of the Fe BCC phase, such as high-temperature self-diffusion even in a pure solid iron, might be responsible for the formation of large-scale anisotropic structures needed to explain the Earth inner core anisotropy,” he says. “The diffusion allows easy texturing of iron in response to any stress.”
The prediction opens the path to understanding the interior of the Earth and eventually to predicting Earth’s future, Belonoshko says. “The ultimate goal of Earth Sciences is to understand the past, present and future of the Earth - and our prediction allows us to do just that.”
David Callahan
Read the paper in Nature Geosciences
Contact Anatoly Belonoshko at anatoly@kth.se or +46 8 790 82 88